Carbon cycle and circular bioeconomy
The main source for new raw materials for circular bioeconomy is the productive biological carbon cycle formed by the biosphere and the atmosphere (Figure 1). Plant biomass represents c. 80 % of the biosphere’s total carbon content (excluding soil carbon), and 2/3 of this biomass is in forests1,2. The biosphere includes also the pedosphere, or soil. Timber is the most versatile and an abundant resource produced by forest ecosystems. Recycled raw materials, instead, come from the “consumersphere” – or human economy. As an example, recycled paper and board products represent more than 50 % of the feedstocks used in all paper and board manufacture. Recycling used textile products and construction lumber are increasingly practiced.
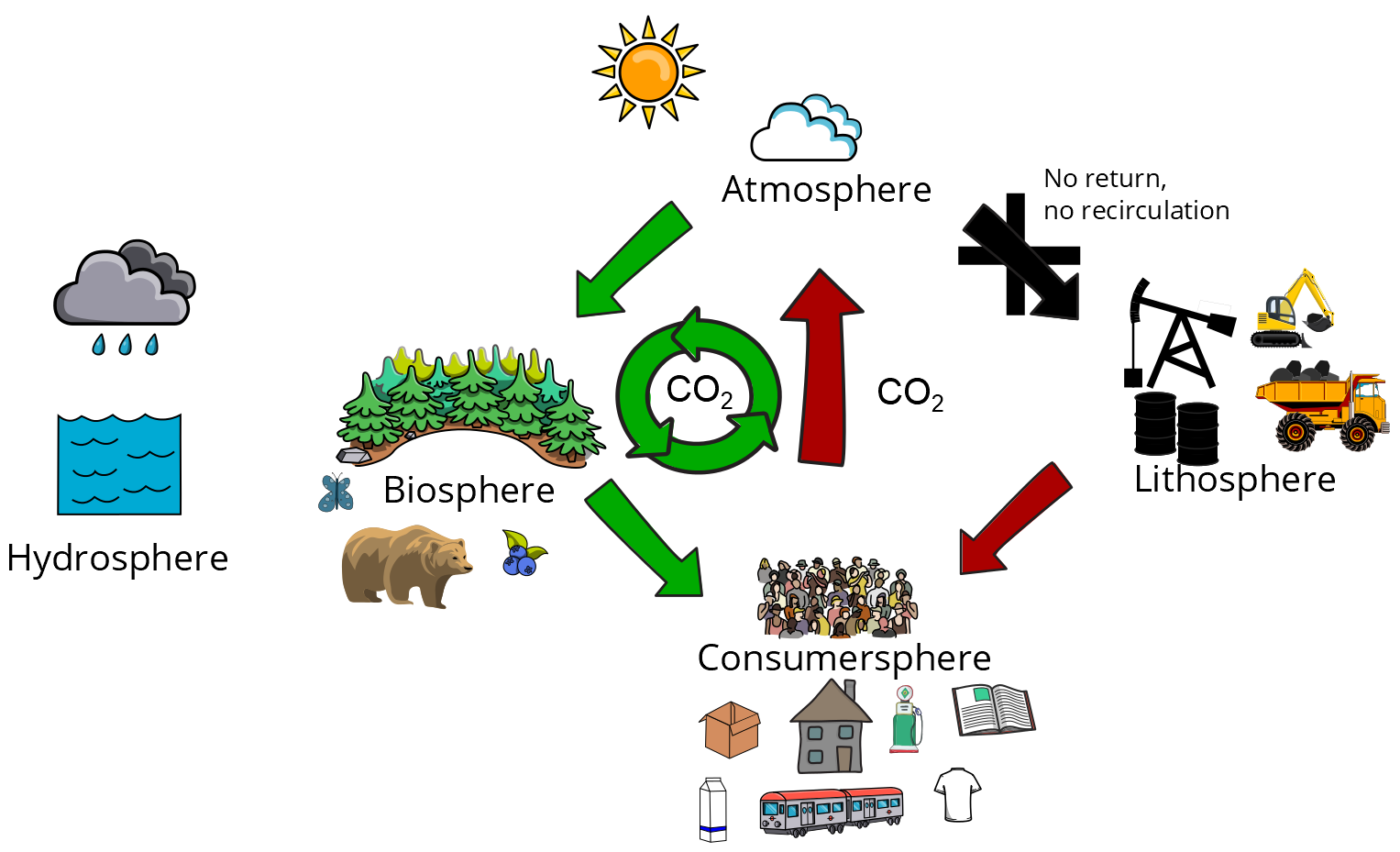
In the forest industry, the main energy source is renewable biomass. At pulp mills, it is lignin, which is dissolved from wood chips during the pulping process, then concentrated and used as liquid biofuel in the mills’ integrated chemical recovery and power plants- so called recovery boilers. Wood bark is utilised as fuel as well. In the saw mill industry, sawdust and other wood residues are the main fuels. Modern, large pulp mills are not only self-sufficient in electric power and steam, but can also provide CO2-neutral electricity to national (and increasingly international) power grids and renewable thermal bioenergy e.g., for a paper or board mill’s process needs or for heating surrounding communities.
Carbon cycles include sinks, storages and fluxes
Global material and energy storages in, and the fluxes between, the biosphere, atmosphere, hydrosphere and lithosphere form the highly complex, dynamic system (Figure 2). The human economy, or the consumersphere, is the rapidly developing newcomer to this system, as compared to the evolution and ages of the other spheres. The human economy provides the system with only small carbon storages but influences carbon fluxes much more strongly.
The term carbon sink refers to growth of a carbon storage. Growing forests provide the carbon cycle with carbon sinks, as carbon accumulates in trees through biosynthesis. When the forest gets old enough, its carbon sink function gradually diminishes. Finally, dying trees remove as much living biomass from the forest ecosystem as growing trees create. Recent results show that accumulation of dead biomass in old forests continues beyond this point 4. Apart from carbon sinks, such old forests provide us with other important ecosystem services, like richness in certain kinds of species and recreation.
Globally, more carbon sinks are needed primarily for two reasons: First, to compensate for past and future CO2 emissions from fossil fuel usage and, secondly, to compensate for deforestation, which is mostly caused by expanding agriculture, but also by illegal logging related to poor forest management 5.
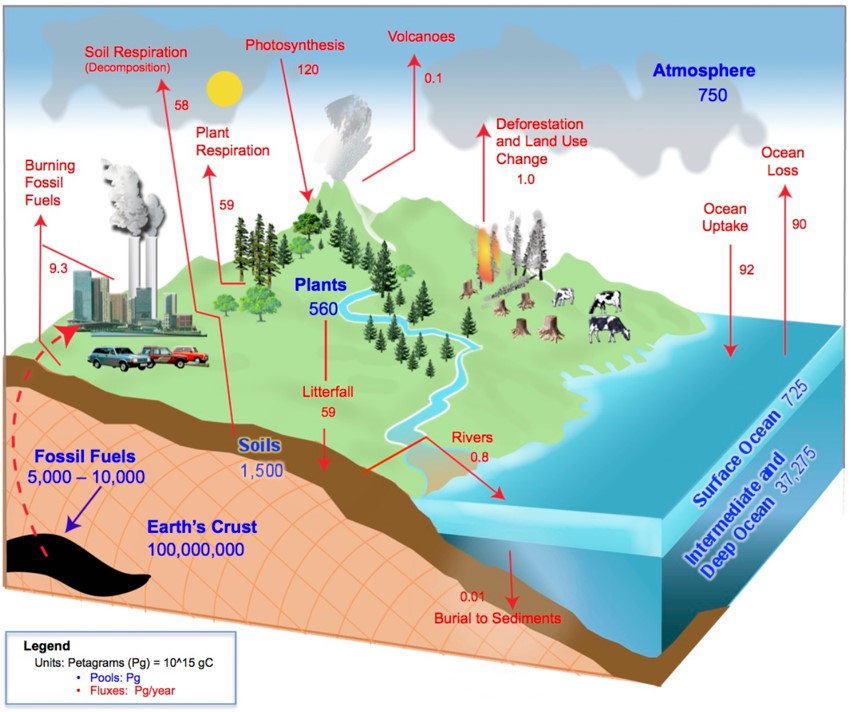
Rotation of carbon in the biological cycle, driven by living organisms, is very fast as compared the geological carbon cycle, which is based on very slow geological processes. Biological and geological carbon cycles are connected to shared carbon storages of the atmosphere and the hydrosphere. The same pools of atmospheric and oceanic CO2 that are utilised by the biosphere are also fed and depleted by natural, slow geological processes (Figure 2.). Apart from these two continuously operating cycles, Earth’s massive non-renewable fossil deposits were formed from plant biomass hundreds to tens of millions of years ago through a slow process, which took millions of years6.
Adding to the abovementioned natural carbon fluxes, burning of fossil fuels (coal, oil and natural gas) generates an anthropogenic CO2 flux, which is an essential part of the human economy, or the consumersphere. No corresponding natural carbon flux exists to return fossil-based CO2 emissions back to the fossil deposits. Thus, reclaiming and recycling this CO2 flux by other means is to be arranged and paid for by the human economy. In line with the user pays principle, a cornerstone of sustainable market economy 7, the cost of recycling fossil CO2 should be included in the prices of fossil fuels. However, from the commercialisation of fossil fuels until now, this cost has been externalised from fossil fuel prices for the coming generations to pay, and the recycling is yet to be arranged.
Nevertheless, a good part of fossil CO2 emissions can be absorbed by the biological carbon cycle and oceans (and much less by the geological carbon cycle). Still, much of it has accumulated in the atmosphere since the discovery of fossil fuels – and is continuing to do so at a rapidly accelerating rate, increasing the atmosphere’s CO2 concentration. For instance, Earth’s heat balance, its climate, the biosphere and oceans carbonate chemistry are sensitive to this increase. Atmospheric CO2 concentration has risen from 280 ppm in 1900 to the current level of 400 ppm (parts per million)6,8.
On the plus side, increased availability of CO2 from air has a fertilising effect on forests and has already increased the biosphere’s biosynthetic capacity considerably 8. However, this effect is not strong enough to absorb all fossil CO2 emissions into the biosphere-atmosphere carbon cycle. On the minus side, rising atmospheric CO2 concentration causes global warming and leads to, e.g., climate change and sea-level rise. Oceans’ major role in the global carbon cycle is indicated in Figure 2., but not discussed in furter detail here.
Both reducing the use of fossil fuels and expanding properly managed production forest area are important, doable and practical ways to enhance sustainable circular bioeconomy.
The importance of forest management
Recent advances in global long-term modelling of forest related carbon storages and fluxes revealed that the contribution of forest management techniques to global carbon balance has been very positive, reducing the cumulative loss in carbon storage due to timber harvesting since 1900 from an estimated 484Gt carbon (if no forest management was practiced in harvested areas) to 92Gt carbon (forest management as it was actually practiced). Largely thanks to well-developed management of production forests in most of the industrialised world, the total contribution of land-use changes, including also deforestation for agriculture and due to unmanaged timber harvesting, is estimated to be only 7 % of total anthropogenic carbon emissions since 1900. Modern forest management techniques were developed to their current level during the last 70 years, and became intensively applied in many parts of the world – and less intensively in many other parts9.
Forest management includes, e.g., planting trees, selecting varieties, optimising stocking (number of trees per hectare) by thinning, controlled fertilising, and other practices that enhance forest growth and its renewal.
As an alternative to the use of well-managed production forests for expanding forest-based bioeconomy, it has been suggested that, e.g., in the EU, such forests could be harvested less and, instead, allow them to act more as carbon sinks for the next 10-30 years, in order to meet regional or national policy targets. For sustainable forest management, this represent short term target setting. Such action would also undermine the targets of bio-economy and global sustainability. As markets of forest-based products are global, this can lead to increased timber harvesting in unmanaged forests elsewhere and, thus, to negative net impact to carbon neutrality on the global scale. Also, investments in forest-based bioeconomy in regions and countries with highly developed and sustainable forestry sectors may reduce, causing an additional, regional negative impact in the longer term10. Considering also global and long-term impacts is of great importance, when setting regional or national targets for achieving carbon neutrality in the shorter perspective.
As the scientists’ awareness of the threats of climate change has been increasingly shared also by the general public, it has caused “climate anxiety”- a natural response to a threat. Gaining knowledge of the carbon cycle and climate change, and contributing to well-planned, practical steps to mitigate it, is the reasonable way to translate climate anxiety to fruitful action. Enhancing forest-based circular bioeconomy provides practical opportunities for doing this.
Further reading
- The Globe Project
- Second State of the Carbon Cycle Report (2018). U.S. Global Change Research Program
- Hetemäki L. et al. (ed.) (2017). Towards a sustainable European forest-based bioeconomy.